Modality
Nuclear Medicine
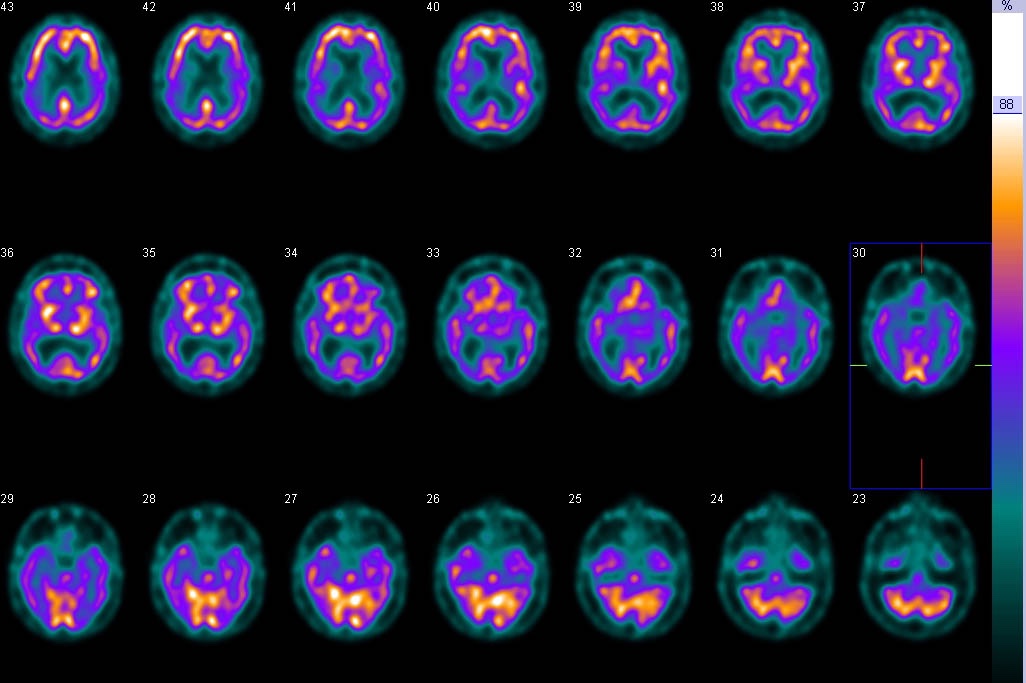
Introduction
Nuclear medicine (or nuclear radiology) is unique within the field of medical imaging. While most images created in radiology are direct depictions of anatomy and structure, nuclear medicine studies are depictions of physiology and function. The studies are specifically designed to take advantage of changes in function that occur with pathologic processes. Functional changes often become apparent earlier than derangement of macroscopic anatomy, making nuclear imaging highly sensitive.
This introduction serves to explain the basis for, and techniques utilized to create nuclear medicine imaging studies and will guide you to an understanding of nuclear imaging. The role that nuclear medicine plays in the rapidly developing field of personalized medicine will also be touched upon.
The tracer principle
The key to understanding nuclear medicine is the realization that when disease occurs, the physiologic processes at the site of disease change. This will be elucidated with the three most commonly performed examinations:
- FDG PET for malignancy evaluation
- Bone scans
- Myocardial perfusion scans.
FDG PET for malignancy evaluation
Nuclear imaging for malignancy exploits the differences in the way the tumor functions relative to normal tissue. The majority of malignancies have excessively high energy demands and meet these by metabolizing glucose. Thus, the glucose uptake by most tumors is markedly elevated compared to background (normal) tissue.
The glucose uptake in the body can be measured and imaged by administering a small dose of a glucose analog labeled with a radiation emitter (fluoro-deoxy glucose or FDG) intravenously and then placing the patient into a scanner (which is a radiation detector). Areas of malignancy will be manifest as sites of increased uptake of FDG. The FDG administered is a “tracer.” It will distribute in a manner similar to glucose but will have no impact on the body: it does not change organ function, is not recognized as a foreign substance and will cause no apparent side effects (other than the uncertain impact that people receive from low doses of radiation). In effect, only a “trace” dose is given.
Bone scans
Bone scans are a depiction of bone turnover. Almost all processes that cause bone injury lead to a reparative process accomplished by increasing bone turnover. These scans are performed by administering a radio-labeled phosphate compound (methylene diphosphonate or MDP) intravenously. The phosphate is incorporated into the skeleton and areas of increased turnover (as occurs with skeletal metastases, fractures or osteomyelitis) are identified with the scanner.
Myocardial perfusion scans
Finally, myocardial perfusion scans are performed principally for the evaluation of blood flow to the left ventricle. The tracers available (including sestamibi and tetrofosmin) are picked up by myocardial mitochondria relative to coronary flow. The state of flow to the heart is typically evaluated during phases of both rest and stress (usually with treadmill exercise or simulated with a vasodilator) for the detection of coronary artery disease.
Isotopes and emissions
An element is defined by its atomic number (number of protons), but may have multiple different isotopes: versions of that element with different numbers of neutrons and, therefore, different atomic weights. For example, carbon (six protons) has multiple isotopes: carbon-11, carbon-12, carbon-14. Some isotopes may be stable and some are radioactive. Some elements have no stable isotopes. Radioactive elements undergo decay events and emit energy. Energy can be emitted in multiple forms, either as energetic particles (e.g., alpha and beta particles) or as photons (having no mass; e.g., x-rays and gamma rays). Only photons are used to create images. Most studies are performed with gamma rays.
The four most commonly used isotopes in clinical nuclear medicine are:
- Technetium-99m - used for a wide variety of study types
- Indium-111 - for tagged white blood cell scans
- Iodine-131/123 - thyroid imaging
- Fluorine-18 - PET imaging
Scanners and techniques
The scanners used in nuclear medicine are, much like Geiger counters, radiation detectors; they emit no radiation. Scanners record the photons being emitted from the patient, who becomes the source of radiation following the administration of the isotope. The emissions can be quantified, localized, and converted into images.
Gamma cameras v PET scanners
Scanners can be grouped into two large, but quite distinct, categories: gamma cameras and PET scanners. They are both radiation (photon) detectors that convert the emissions into images.
Gamma cameras count photons emitted, one at a time. The most commonly used isotope in gamma cameras is technetium-99 metastable or (Tc-99m). PET scanners are used exclusively for studies performed with positron emitting isotopes. (A positron is a beta particle with a positive charge). After emission from a nucleus, it will travel a short distance and then undergo what is known as “annihilation” with an electron. In the annihilation reaction, the mass of the positron and of the electron are converted completely into energy in the form of two photons traveling in opposite directions. The scanner needs to detect both photons simultaneously for it to be counted. The most commonly used isotope in PET scanners is fluorine-18 (F-18).
Planar vs. tomographic images

Simply, planar imaging is a two dimensional representation of a three dimensional object (think chest x-ray).
Some studies are typically done in a planar format (e.g. bones scans). The image on the right is a bone scan of a patient with prostate cancer, anterior view on the left and posterior view on the right. Areas of high radiotracer uptake are black. Multiple areas of intensely increased activity in the axial and appendicular skeleton represent sites of metastases.
A gamma camera can be stationary and acquire planar images, as shown, or it can rotate around the patient and acquire tomographic images (more details on tomographic imaging are on the next page).
Tomographic imaging is a two dimensional representation of a two dimensional object (think slices from a CT). In actuality, CT images do have some thickness, but not much. Tomographic images are created by rotating a gamma camera around the patient while taking pictures and then using a computer to reconstruct the image (hence, “computed tomography”-A CT scanner does this with x-rays).
A gamma camera does the same with the gamma rays emitted by the patient, creating tomographic images known as SPECT: single photon emission computed tomography. A gamma camera can take planar images or tomographic (SPECT) images. Some studies are done exclusively in tomographic format (cardiac perfusion scans). The image displayed here is a dual headed gamma camera. There is a head positioned above the patient table (labeled “1”) and the second head is underneath and obscured by the table. The heads can stay stationary, to acquire planar images, or rotate around the patient to acquire tomographic images. The scanner shown is also a hybrid scanner which combines a CT scanner (the ring labelled "Symbia") and a gamma camera.
Hybrid imaging
Because nuclear studies are depictions of functional processes, the images have low spatial resolution and poor anatomic detail. It is often necessary to view them in conjunction with anatomic studies (like radiographs and CT scans) to provide useful additional localizing information. To improve this process, hybrid cameras were developed, such as the PET-CT. A PET-CT scanner performs both the CT scan and the PET scan, and the hardware for both studies is ‘housed’ in the same unit. This allows the images to be overlain, or “registered.” Following the wide clinical acceptance of PET-CT, gamma cameras were coupled to CT scanners, called SPECT CT (see prior page) to improve anatomic localization of tomographic nuclear images. PET-MRI is now available as well.
The image shown is a PET-CT. This device combines a PET scanner (which acquires tomographic images) and a CT scanner under one housing. Be aware that with PET-CT, there are two sources of radiation exposure to the patient, one from the CT scan, and one from the injected radiotracer.
Terminology & image display

Nuclear images are most commonly depicted in grayscale, although studies can be depicted in color as well.
On grayscale images, by most conventions, areas of high radiotracer uptake are displayed in black, and areas of low uptake are white, with varying intermediate shades of gray. Areas of high uptake are commonly called “hot or radiotracer avid”. Areas of low uptake are called “cold or photopenic”.
The bone scan images shown are from two different time points. That on the left shows a hot spot in the medial left knee (red arrow) due to increased tracer uptake from bone turnover related to osteoarthritis. The patient then had a total knee replacement using a metal prosthesis. The bone scan on the right, done post-operatively, shows a cold spot in the left knee (yellow arrow) corresponding to the metal prosthesis, which cannot take up any tracer.
Indications for nuclear imaging
The scans offered in nuclear medicine are numerous, diverse, and cover all organ systems. Presently, the most commonly performed studies are FDG PET-CT, myocardial perfusion scans and bone scans.
FDG PET-CT
FDG is fluoro-deoxy glucose (a glucose analog labeled with a radioactive isotope of fluorine, F-18). Scanning with FDG gives a depiction of glucose metabolism throughout the body. A majority of malignancies are highly dependent on glucose for energy and avidly pick up this tracer. This includes such common tumors as lung, breast cancer, and lymphoma. FDG PET-CT has proven highly valuable for diagnosis, the evaluation of treatment response and the monitoring of recurrence. The images shown are from a PET-CT in a smoker with an indeterminate lung nodule. The coronal PET image (right) shows intense activity in the right upper lobe corresponding to a nodule demonstrated on the accompanying CT (left). This indicates the presence of markedly increased glucose metabolism, consistent with (and subsequently biopsy proven to be) lung cancer. (Also note the intense tracer uptake by the myocardium, since cardiac muscle can utilize glucose for metabolism).
Myocardial perfusion scans
Myocardial perfusion imaging (MPI) is a method to map blood flow to the left ventricle. The most commonly used tracers are Tc-99m sestamibi, Tc-99m tetrofosmin and thallium-201 chloride. Scans are usually performed for the detection of ischemic heart disease, assessment of the significance of a coronary stenosis and the follow up of patients after therapy. Most often patients will be scanned in two stages: during rest and under stress (induced by either exercise or simulated with a vasodilator). Areas of the myocardium with poor radiotracer uptake are called ‘defects’. Those that occur during stress typically represent ischemia and those found at both stress and rest indicate myocardial infarction.
The myocardial perfusion scan shows left ventricular radiotracer uptake during stress (treadmill exercise, top row) and at rest (bottom row). Stress images show reduced tracer uptake in the lateral wall (arrow) indicating stenosis of the left circumflex coronary artery.
Bone scans

Bone scans, or skeletal scintigraphy are performed with a radiolabeled phosphate analog (most commonly Tc-99m MDP). Bone turnover is a continual process; pathology that causes bone injury will lead to bone repair and the increased incorporation of phosphates and MDP. The processes typically evaluated with bone scans are skeletal metastatic disease, fractures and osteomyelitis. The most common indications are the staging and follow up of patients with prostate and breast cancer.
Shown on the right is the same bone scan shown earlier in this module, demonstrating multifocal "hot spots" due to prostate cancer metastasis to the spine, ribs and pelvis.